In population genetics, gene flow (also known as gene migration) is the transfer of alleles or genes from one population to another.
Migration into or out of a population may be responsible for a marked change in allele frequencies (the proportion of members carrying a particular variant of a gene). Immigration may also result in the addition of new genetic variants to the established gene pool of a particular species or population.
There are a number of factors that affect the rate of gene flow between different populations. One of the most significant factors is mobility, as greater mobility of an individual tends to give it greater migratory potential. Animals tend to be more mobile than plants, although pollen and seeds may be carried great distances by animals or wind.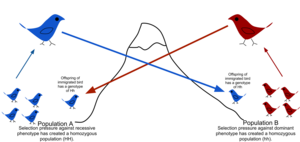
Gene flow is the transfer of alleles from one population to another population through immigration of individuals. In this example, one of the birds from population A immigrates to population B, which has fewer of the dominant alleles, and through mating incorporates its alleles into the other population.
Maintained gene flow between two populations can also lead to a combination of the two gene pools, reducing the genetic differentiation between the two groups. It is for this reason that gene flow strongly acts against speciation, by recombining the gene pools of the groups, and thus, repairing the developing differences in genetic variation that would have led to full speciation and creation of daughter species.
For example, if a species of grass grows on both sides of a highway, pollen is likely to be transported from one side to the other and vice versa. If this pollen is able to fertilize the plant where it ends up and produce viable offspring, then the alleles in the pollen have effectively been able to move from the population on one side of the highway to the other.
Barriers to Gene Flow
Physical barriers to gene flow are usually, but not always, natural. They may include impassable mountain ranges, oceans, or vast deserts. In some cases, they can be artificial, man-made barriers, such as the Great Wall of China, which has hindered the gene flow of native plant populations. One of these native plants, Ulmus pumila, demonstrated a lower prevalence of genetic differentiation than the plants Vitex negundo, Ziziphus jujuba,Heteropappus hispidus, and Prunus armeniaca whose habitat is located on the opposite side of the Great Wall of China where Ulmus pumila grows. This is because Ulmus pumilahas wind-pollination as its primary means of propagation and the latter-plants carry out pollination through insects. Samples of the same species which grow on either side have been shown to have developed genetic differences, because there is little to no gene flow to provide recombination of the gene pools.
Barriers to gene flow need not always be physical. Species can live in the same environment, yet show very limited gene flow due to limited hybridization or hybridization yielding unfit hybrids.
Female choice can also play a role in hindering gene flow. Asymmetric recognition of local and nonlocal songs has been found between two populations of black-throated blue warblers in the United States, one in the northern United States (New Hampshire) and the other in the southern United States (North Carolina). Males in the northern population respond strongly to the local male songs but relatively weakly to the nonlocal songs of southern males. In contrast, southern males respond equally to both local and nonlocal songs. The fact that northern males exhibit differential recognition indicates that northern females tend not to mate with “heterospecific” males from the south; thus it is not necessary for the northern males to respond strongly to the song from a southern challenger. A barrier to gene flow exists from South to North as a result of the female preference.
Gene Flow in HumansGene flow has been observed in humans. For example, in the United States, gene flow was observed between a white European population and a black West African population, which were recently brought together. In West Africa, where malaria is prevalent, the Duffy antigen provides some resistance to the disease, and this allele is thus present in nearly all of the West African population. In contrast, Europeans have either the allele Fya or Fyb, because malaria is almost non-existent. By measuring the frequencies of the West African and European groups, scientists found that the allele frequencies became mixed in each population because of movement of individuals. It was also found that this gene flow between European and West African groups is much greater in the Northern U.S. than in the South.
Gene Flow Between Species
Horizontal gene transfer (HGT) refers to the transfer of genes between organisms, either through hybridization, antigenic shift, or reassortment is sometimes an important source of genetic variation. Viruses can transfer genes between species. Bacteria can incorporate genes from other dead bacteria, exchange genes with living bacteria, and can exchange plasmids across species boundaries.[5] "Sequence comparisons suggest recent horizontal transfer of many genes among diverse species including across the boundaries of phylogenetic "domains". Thus determining the phylogenetic history of a species can not be done conclusively by determining evolutionary trees for single genes."
Biologist Gogarten suggests "the original metaphor of a tree no longer fits the data from recent genome research". Biologists [should] instead use the metaphor of a mosaic to describe the different histories combined in individual genomes and use the metaphor of an intertwined net to visualize the rich exchange and cooperative effects of horizontal gene transfer.
"Using single genes as phylogenetic markers, it is difficult to trace organismal phylogeny in the presence of HGT. Combining the simple coalescence model of cladogenesis with rare HGT events suggest there was no single last common ancestor that contained all of the genes ancestral to those shared among the three domains of life. Each contemporary molecule has its own history and traces back to an individual molecule cenancestor. However, these molecular ancestors were likely to be present in different organisms at different times."
Genetic PollutionNaturally-evolved, region-specific species can be threatened with extinction through genetic pollution, potentially causing uncontrolled hybridization, introgression and genetic swamping. These processes can lead to homogenization or replacement of local genotypes as a result of either a numerical and/or fitness advantage of introduced plant or animal. Nonnative species can threaten native plants and animals with extinction by hybridization and introgression either through purposeful introduction by humans or through habitat modification, bringing previously isolated species into contact. These phenomena can be especially detrimental for rare species coming into contact with more abundant ones. Interbreeding between the species can cause a 'swamping' of the rarer species' gene pool, creating hybrids that supplant the native stock. The extent of this phenomenon is not always apparent from outward appearance alone. While some degree of gene flow occurs in the course of normal evolution, hybridization with or without introgression may threaten a rare species' existence. For example, the Mallard is an abundant species of duck that interbreeds readily with a wide range of other ducks and poses a threat to the integrity of some species.
References
- ^ a b c Su H, Qu LJ, He K, Zhang Z, Wang J, Chen Z, Gu H (March 2003). "The Great Wall of China: a physical barrier to gene flow?". Heredity 90 (3): 212–9. doi:10.1038/sj.hdy.6800237, PMID 12634804.
- ^ Colbeck, G.J.; Sillett, T.S.; Webster, M.S. (2010). "Asymmetric discrimination of geographical variation in song in a migratory passerine". Animal Behaviour 80 (2): 311–318. doi:10.1016/j.anbehav.2010.05.013.
- ^ "Brain & Ecology Deep Structure Lab". Brain & Ecology Comparative Group. Brain & Ecology Deepstruc. System Co., Ltd. 2010. Retrieved March 13, 2011.
- ^ http://webcache.googleusercontent.com/search?q=cache:tpICVNWaTbgJ:non.fiction.org/lj/community/ref_courses/3484/enmicro.pdf+sex+evolution+%22Horizontal+gene+transfer%22+-human+Conjugation+RNA+DNA&hl=en.
- ^ http://www2.nau.edu/~bah/BIO471/Reader/Pennisi_2003.pdf.
- ^ http://opbs.okstate.edu/~melcher/MG/MGW3/MG334.html.
- ^ Horizontal Gene Transfer - A New Paradigm for Biology (from Evolutionary Theory Conference Summary), Esalen Center for Theory & Research.
- ^ http://web.uconn.edu/gogarten/articles/TIG2004_cladogenesis_paper.pdf.
- ^ Mooney, H. A.; Cleland, E. E. (2001). "The evolutionary impact of invasive species". PNAS 98 (10): 5446–5451, doi:10.1073/pnas.091093398 PMC 33232 PMID 11344292.
- ^ Aubry, C.; Shoal, R.; Erickson, V. (2005). "Glossary". Grass cultivars: their origins, development, and use on national forests and grasslands in the Pacific Northwest. Corvallis, OR: USDA Forest Service; Native Seed Network (NSN), Institute for Applied Ecology.
- Wikipedia